Home>Misc>Featured>What Is The Primary Energy Source During High-Intensity Exercise
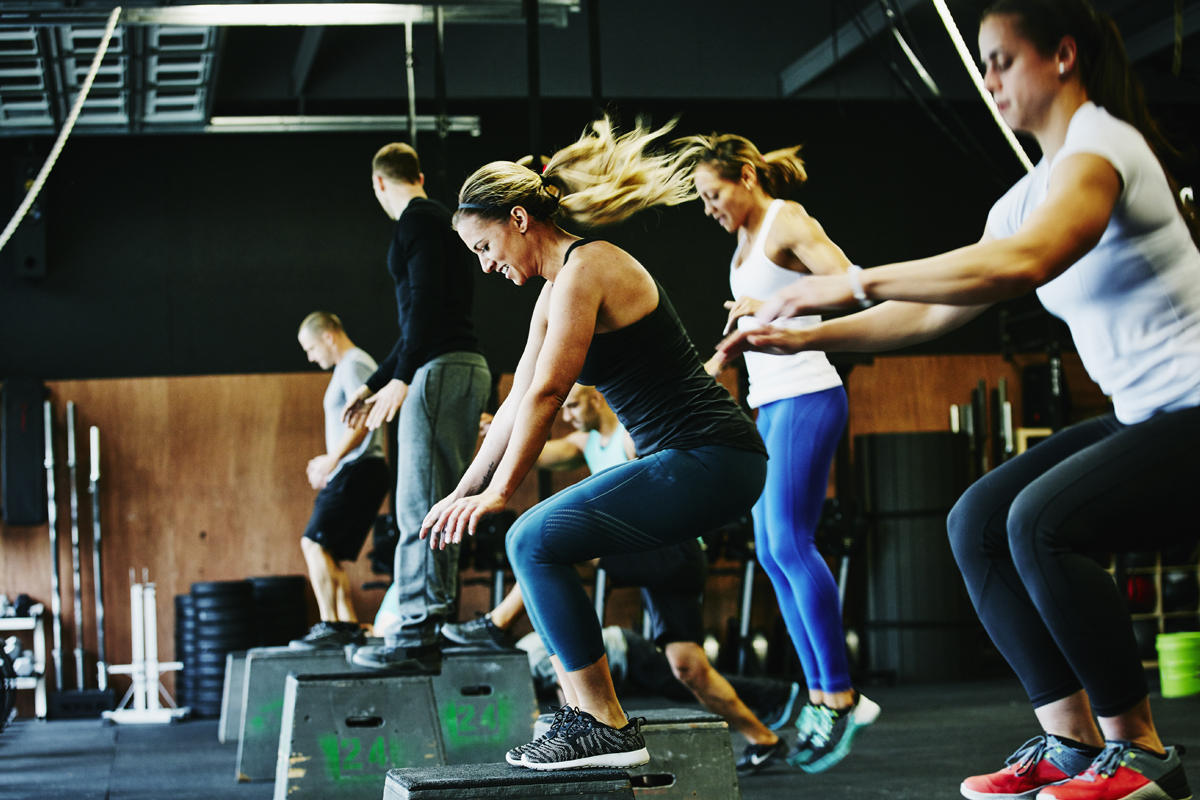
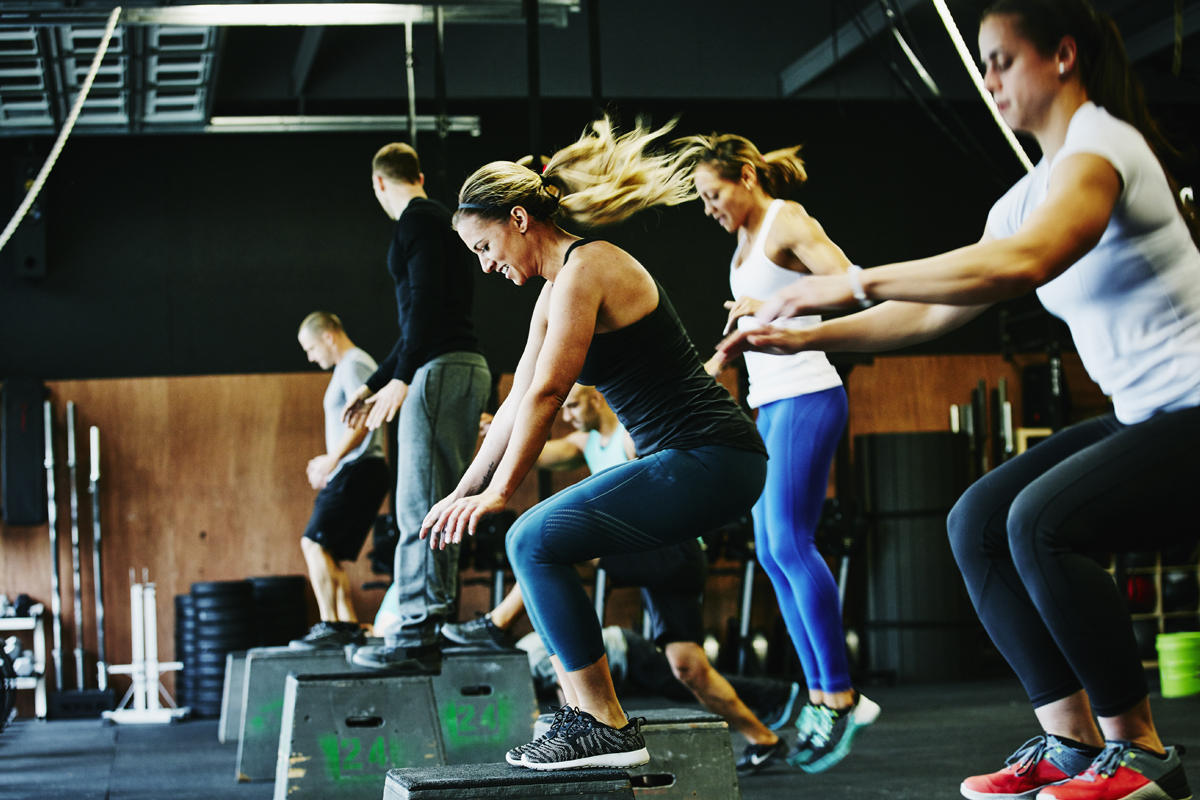
Featured
What Is The Primary Energy Source During High-Intensity Exercise
Modified: August 19, 2023
Discover the featured primary energy source utilized during high-intensity exercise and how it impacts your performance. Enhance your workout efficiency with expert insights.
Introduction
When engaging in high-intensity exercise, our bodies rely on a complex interplay of energy systems to support the increased demands placed on our muscles. Understanding these energy systems is crucial for optimizing performance and achieving our fitness goals.
High-intensity exercise is characterized by short bursts of intense activity, such as sprinting or weightlifting, that push our bodies to their limits. During these intense bouts, our muscles require a significant amount of energy to contract and perform the necessary movements. The primary energy source utilized during high-intensity exercise differs depending on the duration and intensity of the activity.
In this article, we will explore the three main energy systems involved in high-intensity exercise: the ATP-PCr energy system, the anaerobic glycolysis energy system, and the aerobic energy system. We will delve into the contribution of each energy system during high-intensity exercise and discuss the dominant energy system at different exercise intensities. Additionally, we will touch on the factors that influence energy system utilization and how training adaptations can enhance energy system efficiency.
By the end of this article, you will have a comprehensive understanding of the primary energy sources utilized during high-intensity exercise and the importance of optimizing energy system utilization for peak performance.
Definition of High-Intensity Exercise
High-intensity exercise refers to physical activities that involve short bursts of intense effort and push our bodies to maximum exertion. It is characterized by its demanding nature, where individuals work at or near their maximum capacity for a limited duration of time. This type of exercise has gained popularity for its ability to deliver efficient and effective results in a shorter period.
High-intensity exercise can take various forms, such as sprinting, interval training, CrossFit, circuit training, and heavy weightlifting. These activities elevate heart rate and breathing intensity, rapidly taxing the body’s energy systems to meet the increased demand. Compared to traditional moderate-intensity exercises like jogging or cycling, high-intensity exercise requires individuals to push beyond their comfort zones and reach their anaerobic threshold.
The duration of high-intensity exercise can vary depending on the specific activity and individual fitness level. Typically, it lasts anywhere from a few seconds to a few minutes, with short recovery intervals between bouts of work. This pattern of intense effort followed by brief rest allows individuals to sustain a high level of performance throughout the workout session.
High-intensity exercise offers numerous benefits, including improved cardiovascular fitness, increased muscle strength and power, enhanced fat burning, and improved overall athletic performance. It is an effective way to challenge the body and elicit adaptations that lead to physical improvements in strength, endurance, and body composition.
It is important to note that high-intensity exercise is not suitable for everyone, especially individuals with pre-existing health conditions or those who are new to exercise. Prior consultation with a healthcare professional or fitness expert is recommended to determine if high-intensity exercise is appropriate and to create a suitable workout plan tailored to individual needs and goals.
Overview of Energy Systems
In order to fuel our muscles during high-intensity exercise, our body turns to three main energy systems: the ATP-PCr energy system, the anaerobic glycolysis energy system, and the aerobic energy system. Understanding how these energy systems work is crucial for optimizing performance and fueling our workouts effectively.
The ATP-PCr energy system, also known as the phosphagen system, provides immediate energy for high-intensity activities lasting up to 10 seconds. It relies on the breakdown of phosphocreatine (PCr) to rapidly resynthesize adenosine triphosphate (ATP), the energy currency of our cells. This system is highly efficient but has limited energy stores, depleting rapidly during intense exercise.
When high-intensity exercise continues beyond the capacity of the ATP-PCr system, the anaerobic glycolysis energy system kicks in. This system utilizes stored glucose in our muscles and liver to produce ATP. Anaerobic glycolysis does not require oxygen, making it a fast and efficient way to produce energy. However, it produces lactic acid as a byproduct, leading to the accumulation of hydrogen ions and a drop in pH, causing muscle fatigue.
For prolonged high-intensity exercise or endurance activities, the aerobic energy system becomes the dominant supplier of energy. This system utilizes oxygen to break down carbohydrates, fats, and even protein to produce ATP. The aerobic system provides a steady supply of energy but is slower in its production compared to the anaerobic systems. It becomes increasingly important as exercise duration or intensity increases.
It is important to note that our energy systems do not work independently but rather work in a coordinated manner to meet the demands of the activity. The contribution of each energy system during high-intensity exercise depends on factors such as exercise intensity, duration, individual fitness level, and available fuel sources.
Understanding the intricacies of these energy systems allows us to develop training strategies that optimize energy production and utilization. It also helps us understand how different types of exercise and training modalities target specific energy systems, allowing us to tailor our workouts to our goals and athletic pursuits.
ATP-PCr Energy System
The ATP-PCr energy system, also known as the phosphagen system, is the primary energy system utilized during high-intensity activities lasting up to 10 seconds, such as a sprint or a heavy lift. It is the fastest way to produce ATP, providing immediate energy for explosive movements.
This energy system relies on the breakdown of phosphocreatine (PCr) to rapidly resynthesize adenosine triphosphate (ATP), the energy currency of our cells. PCr stores are limited in our muscles, and they deplete quickly during intense exercise. As a result, the ATP-PCr system is most effective for short bursts of maximal effort.
When we initiate a high-intensity exercise, such as sprinting, the ATP stores in our muscles are quickly depleted. In response, the enzyme creatine kinase breaks down PCr, releasing a phosphate group that binds with adenosine diphosphate (ADP) to reform ATP. This process is anaerobic and does not require oxygen, providing energy rapidly without the need for oxygen delivery systems like the cardiovascular system.
The ATP-PCr system is highly efficient because it does not rely on any intermediate steps or chemical reactions. The process is direct and instantaneous, making it ideal for quick, explosive movements. However, due to the limited stores of PCr, the ATP-PCr system can only meet the energy demands for a short period before it becomes exhausted.
During high-intensity exercise, the reliance on the ATP-PCr system diminishes as the duration and intensity of the activity increase. As PCr stores deplete, the body must turn to other energy systems to sustain ATP production. This shift typically occurs around the 10 to 15-second mark of intense exercise.
Training interventions that target the ATP-PCr system can enhance its efficiency. Short, intense bursts of exercise that create a demand for ATP-PCr utilization, followed by adequate rest and recovery, can stimulate adaptations such as increased PCr storage, improved enzyme activity, and enhanced ATP production capacity. These adaptations result in improved performance in high-intensity exercise and enable individuals to maintain a high level of power and intensity for longer durations.
In summary, the ATP-PCr energy system is the primary source of energy during high-intensity activities lasting up to 10 seconds. It relies on the breakdown of PCr to rapidly resynthesize ATP, providing immediate energy for explosive movements. While highly efficient, this energy system has limited stores and becomes depleted quickly, necessitating the reliance on other energy systems for sustained high-intensity exercise.
Anaerobic Glycolysis Energy System
When high-intensity exercise continues beyond the capacity of the ATP-PCr system, the anaerobic glycolysis energy system becomes the primary source of energy. This energy system allows for the breakdown of stored glucose in our muscles and liver to produce adenosine triphosphate (ATP) without the need for oxygen.
The anaerobic glycolysis energy system is characterized by the utilization of glycogen, a stored form of glucose, as the primary fuel source. Glycogen is broken down through a series of chemical reactions, resulting in the production of ATP. Unlike aerobic metabolism, this process does not require oxygen and is therefore faster, making it well-suited for high-intensity activities lasting several minutes.
However, the breakdown of glycogen through anaerobic glycolysis also produces a byproduct: lactic acid. As exercise intensity increases, the production of lactic acid increases as well. The accumulation of lactic acid in muscle tissue causes a drop in pH, leading to fatigue and a burning sensation in the muscles.
The anaerobic glycolysis system provides a quick source of energy during high-intensity exercise, but it is not sustainable for long durations due to the limited glycogen stores in muscles and liver. Once these stores are depleted, the energy production rate decreases, leading to a decline in performance.
Training interventions can improve the efficiency of the anaerobic glycolysis energy system. High-intensity interval training (HIIT) and repeated short sprints, for example, can enhance the capacity to produce ATP through anaerobic glycolysis. This type of training stimulates adaptations such as increased glycogen storage, improved enzyme activity, and enhanced lactate clearance, allowing individuals to perform at a high level for longer durations before fatigue sets in.
It is worth noting that while the anaerobic glycolysis system is efficient for producing ATP in the absence of oxygen, it is not as efficient as aerobic metabolism. As a result, the reliance on the anaerobic glycolysis system during high-intensity exercise is associated with the build-up of metabolic byproducts and a greater overall energy cost compared to aerobic metabolism.
In summary, the anaerobic glycolysis energy system is a key contributor during high-intensity exercise lasting several minutes. It breaks down glycogen to produce ATP without the need for oxygen, providing a quick and efficient source of energy. However, the production of lactic acid as a byproduct leads to fatigue and limits the duration of sustainable high-intensity exercise.
Aerobic Energy System
The aerobic energy system, also known as oxidative metabolism, is the primary energy system responsible for fueling prolonged high-intensity exercise and endurance activities. This energy system utilizes oxygen to break down carbohydrates, fats, and even proteins to produce adenosine triphosphate (ATP), the energy currency of our cells.
During high-intensity exercise, the aerobic energy system becomes increasingly important as the duration or intensity of the activity increases. While the anaerobic energy systems provide quick bursts of energy, they are limited in their duration and efficiency. In contrast, the aerobic energy system is more sustainable and provides a steady supply of ATP, making it crucial for endurance activities that last beyond a few minutes.
The breakdown of carbohydrates and fats through aerobic metabolism occurs in the mitochondria of our cells. These organelles act as powerhouses, using oxygen to convert glucose, glycogen, and stored fat into ATP. The process is slower than anaerobic metabolism but highly efficient, yielding a large amount of ATP for sustained exercise.
The beauty of the aerobic energy system is its ability to utilize a variety of fuel sources. During low-intensity exercise or rest periods, the system primarily relies on fat as the fuel source. As exercise intensity increases, carbohydrates become the main fuel source due to their faster rate of ATP production. Proteins can also be broken down and used as fuel, but they are typically a minor contributor unless glycogen stores are depleted.
While the aerobic energy system is highly efficient, there is a lag time between the onset of exercise and the production of ATP. This lag time is known as the oxygen deficit, during which the body relies on anaerobic energy systems to bridge the gap until aerobic metabolism can fully meet the demand for ATP production. This is why the first few minutes of high-intensity exercise may feel challenging before a steady state is reached.
Training interventions that target the aerobic energy system can enhance its efficiency. Activities such as long-distance running, cycling, and swimming that promote sustained exercise at moderate intensities can stimulate adaptations that improve oxygen transport, mitochondrial density, and enzyme activity. These adaptations result in improved endurance and the ability to sustain high-intensity exercise for longer durations.
In summary, the aerobic energy system is the primary source of energy during prolonged high-intensity exercise and endurance activities. It relies on oxygen to break down carbohydrates, fats, and proteins, providing a sustainable supply of ATP. While slower than anaerobic energy systems, the aerobic energy system is highly efficient and can be enhanced through training interventions focused on endurance and moderate-intensity exercise.
Contribution of Energy Systems during High-Intensity Exercise
During high-intensity exercise, our body relies on a combination of energy systems to meet the increased energy demands. The contribution of each energy system depends on factors such as exercise intensity, duration, individual fitness level, and available fuel sources.
In the initial seconds of high-intensity exercise, the ATP-PCr energy system takes center stage. This system provides immediate energy for explosive movements through the breakdown of phosphocreatine (PCr) to regenerate adenosine triphosphate (ATP). It is the primary energy system utilized during activities lasting up to 10 seconds, such as a powerful jump or a quick sprint.
As the duration and intensity of the exercise continue to increase, the contribution of the ATP-PCr system diminishes, and the anaerobic glycolysis energy system becomes more prominent. This system utilizes stored glucose in the muscles and liver to produce ATP through the breakdown of glycogen. It is especially important during high-intensity activities lasting several minutes, like a set of intense weightlifting or a series of short sprints.
While anaerobic glycolysis is efficient in providing ATP without the need for oxygen, it produces lactic acid as a byproduct. As lactic acid accumulates, it can lead to muscle fatigue and a decline in performance. The aerobic energy system plays a critical role in clearing lactic acid and providing sustained energy during longer periods of high-intensity exercise.
The aerobic energy system utilizes oxygen to break down carbohydrates, fats, and even proteins in the mitochondria of our cells, producing ATP as a result. This system becomes increasingly important as exercise duration increases, providing a steady supply of energy. It is the primary energy system utilized during endurance activities such as long-distance running, cycling, or swimming.
The relative contribution of each energy system during high-intensity exercise varies depending on individual factors and exercise characteristics. Higher exercise intensities will rely more on anaerobic energy systems, while lower intensities will draw on the aerobic energy system to a greater extent. Individual fitness level and training adaptations also influence the efficiency and contribution of each energy system.
Understanding the contribution of energy systems during high-intensity exercise is crucial for designing effective training programs. Tailoring workouts to target specific energy systems can optimize performance and improve overall fitness. Combining activities that target both anaerobic and aerobic energy systems, such as interval training, can enhance energy production and utilization, leading to improved endurance and power.
In summary, the contribution of energy systems during high-intensity exercise varies depending on exercise intensity, duration, individual fitness level, and fuel availability. The ATP-PCr energy system is prominent in short bursts of intense activity, while anaerobic glycolysis becomes more significant during longer bouts. The aerobic energy system sustains energy production during endurance activities. Understanding these contributions allows us to design effective training programs and optimize performance.
Dominant Energy System during High-Intensity Exercise
The dominant energy system during high-intensity exercise depends on the intensity, duration, and specific demands of the activity. While all three energy systems – ATP-PCr, anaerobic glycolysis, and aerobic metabolism – contribute to energy production, their relative contributions vary.
In short-duration, maximal-intensity activities lasting up to 10 seconds, the ATP-PCr energy system is the dominant source of energy. It provides the immediate burst of energy needed for explosive movements, such as a sprint or a heavy lift. This energy system relies on the breakdown of phosphocreatine to rapidly replenish ATP, but its stores are limited, depleting rapidly during intense exercise.
As the duration of high-intensity exercise increases beyond the capacity of the ATP-PCr system, the dominant energy system transitions to anaerobic glycolysis. This system utilizes stored glycogen to produce ATP through the breakdown of glucose. Anaerobic glycolysis does not require oxygen and is efficient in providing energy for intense activities lasting several minutes, such as a series of short sprints or a set of heavy resistance exercises.
For high-intensity exercise lasting beyond a few minutes or endurance activities, the dominance shifts to the aerobic energy system. This system relies on oxygen to break down carbohydrates, fats, and even proteins to produce ATP. It provides a sustained and steady supply of energy, making it crucial for activities like long-distance running or cycling.
While the aerobic energy system becomes the dominant source of energy during prolonged high-intensity exercise, the contribution from anaerobic glycolysis remains significant. Even during endurance activities, the body continues to rely on the anaerobic energy systems to a certain extent to provide rapid ATP production during times of high-intensity efforts within the overall endurance exercise.
It is important to note that the relative dominance of energy systems can vary among individuals based on factors such as training background, fitness level, and genetics. Highly trained athletes, for example, may have a more efficient aerobic energy system and a higher anaerobic threshold, allowing them to sustain higher intensities for longer durations before relying heavily on anaerobic energy systems.
Training adaptations can also impact the dominant energy system during high-intensity exercise. Endurance training, for instance, can enhance the efficiency of the aerobic energy system, allowing individuals to maintain a higher intensity for longer periods before relying on anaerobic metabolism. On the other hand, training interventions targeting the anaerobic energy systems, such as interval training, can increase their capacity and delay the shift to aerobic metabolism.
In summary, the dominant energy system during high-intensity exercise is determined by the intensity, duration, and specific demands of the activity. The ATP-PCr energy system is dominant for short, maximal-intensity bursts, while anaerobic glycolysis becomes prominent as the duration increases. For prolonged high-intensity exercise, the aerobic energy system becomes the primary source of energy, although the contribution of anaerobic energy systems remains significant. Training adaptations can influence the dominance of specific energy systems, allowing individuals to improve performance and optimize energy production during high-intensity exercise.
Factors Affecting Energy System Utilization
Several factors influence the utilization of energy systems during high-intensity exercise. Understanding these factors is essential for optimizing performance and designing effective training programs. The key factors affecting energy system utilization include exercise intensity, duration, individual fitness level, and available fuel sources.
Exercise intensity plays a significant role in determining which energy system is primarily utilized. Higher intensities, such as near-maximal efforts, rely more on the anaerobic energy systems – ATP-PCr and anaerobic glycolysis. Lower intensities are more reliant on the aerobic energy system, allowing for sustained energy production over a longer duration.
Exercise duration is another critical factor. For short bursts of high-intensity exercise lasting up to 10 seconds, the ATP-PCr system dominates. As the duration increases beyond the capacity of the ATP-PCr system, the anaerobic glycolysis system becomes more prominent. Prolonged high-intensity exercise requires a shift towards the aerobic energy system, which is better equipped to sustain energy production over an extended period.
Individual fitness level also influences energy system utilization. Well-trained individuals generally have a more efficient aerobic energy system, allowing them to sustain higher intensities for longer durations before relying heavily on anaerobic metabolism. They may also exhibit a higher anaerobic threshold, delaying the transition to anaerobic energy systems even during prolonged high-intensity exercise.
The availability of fuel sources, such as glycogen (from carbohydrates) and stored fats, also impacts energy system utilization. The depletion of glycogen stores within the muscles and liver can shift the reliance towards anaerobic metabolism earlier, accelerating fatigue. Conversely, individuals with higher glycogen stores and better fat utilization can prolong the reliance on the aerobic energy system.
It’s important to note that the fuel sources utilized during high-intensity exercise can be influenced by dietary and nutritional strategies. Proper carbohydrate intake prior to exercise can enhance glycogen stores, while a diet rich in healthy fats can enhance fat utilization during aerobic metabolism. These nutritional strategies can optimize energy system utilization and improve performance during high-intensity exercise.
Training adaptations also play a crucial role in energy system utilization. Endurance training, which involves sustained exercise at moderate intensities, can enhance the efficiency of the aerobic energy system. This allows individuals to maintain higher intensities for longer durations without transitioning to anaerobic metabolism. On the other hand, high-intensity interval training (HIIT) and other anaerobic-focused training methods can target and improve the capacity of the anaerobic energy systems.
In summary, multiple factors influence energy system utilization during high-intensity exercise. Exercise intensity and duration, individual fitness level, available fuel sources, dietary strategies, and training adaptations all come into play. Understanding these factors allows individuals to optimize their training, fueling, and performance, providing the necessary energy for high-intensity exercise and achieving their fitness goals.
Training Adaptations and Energy System Efficiency
Training adaptations play a crucial role in improving the efficiency and function of the energy systems involved in high-intensity exercise. Regular training can elicit specific physiological changes, enhancing energy system utilization and improving overall performance. Let’s explore the training adaptations that lead to enhanced energy system efficiency.
Endurance training, which involves sustained exercise at moderate intensities, is a powerful stimulus for improving the aerobic energy system. This type of training increases the efficiency of oxygen delivery to the muscles, improves mitochondrial density, and enhances enzyme activity involved in aerobic metabolism. These adaptations result in a greater capacity for aerobic energy production and utilization during high-intensity exercise. As a result, individuals can sustain high-intensity efforts for longer durations before relying on anaerobic energy systems.
High-intensity interval training (HIIT) specifically targets the anaerobic energy systems, including the ATP-PCr and anaerobic glycolysis systems. HIIT involves short bursts of near-maximal effort followed by brief recovery periods. This type of training stimulates adaptations such as increased phosphocreatine storage, improved anaerobic enzyme activity, and enhanced lactate clearance. These adaptations improve the efficiency of the ATP-PCr and anaerobic glycolysis systems, allowing for sustained high-intensity efforts and delaying the onset of fatigue.
Resistance training, commonly known as weightlifting or strength training, also plays a role in energy system adaptations. While resistance training primarily targets muscular strength and hypertrophy, it indirectly impacts energy system efficiency. Increased muscular strength allows individuals to exert more force and power during high-intensity exercises, enhancing the demand for ATP production. This can lead to improvements in the efficiency and capacity of the ATP-PCr and anaerobic glycolysis systems.
The metabolic adaptations resulting from training can also improve the utilization of fuel sources during high-intensity exercise. Endurance training promotes enhanced fat utilization as an energy source, which can be beneficial for prolonged endurance activities. HIIT, on the other hand, improves the capacity to rapidly break down glycogen and utilize glucose, enhancing anaerobic glycolysis efficiency. Optimal nutrition and dietary strategies, alongside training, can further enhance these metabolic adaptations.
It is essential to note that the specific adaptations and their magnitude may vary among individuals based on factors such as starting fitness level, genetics, and training program design. Nevertheless, regular and consistent training that targets the energy systems involved in high-intensity exercise can lead to significant improvements in energy system efficiency and overall performance.
In summary, training adaptations play a critical role in enhancing energy system efficiency during high-intensity exercise. Endurance training improves the aerobic energy system, HIIT enhances the anaerobic energy systems, and resistance training indirectly impacts energy system function. These adaptations include improvements in oxygen delivery, mitochondrial density, enzyme activity, phosphocreatine storage, and metabolic utilization of fuel sources. By tailoring training programs to target specific energy systems, individuals can optimize energy production and utilization, leading to improved performance and achieving their desired fitness goals.
Conclusion
Understanding the interplay of energy systems during high-intensity exercise is essential for optimizing performance and achieving fitness goals. High-intensity exercise relies on three primary energy systems: ATP-PCr, anaerobic glycolysis, and aerobic metabolism. The contribution of each energy system depends on factors such as exercise intensity, duration, individual fitness level, and available fuel sources.
The ATP-PCr energy system provides immediate energy for short bursts of intense exercise, relying on the breakdown of phosphocreatine to regenerate ATP. The anaerobic glycolysis system becomes prominent during longer bouts of high-intensity exercise, utilizing stored glucose to produce ATP without the need for oxygen. The aerobic energy system plays a critical role in providing sustained energy during prolonged high-intensity exercise, using oxygen to break down carbohydrates, fats, and proteins to produce ATP.
Several factors affect energy system utilization, including exercise intensity, duration, individual fitness level, and available fuel sources. Training adaptations, such as endurance training, high-intensity interval training (HIIT), and resistance training, can enhance energy system efficiency. These adaptations include improvements in oxygen delivery, mitochondrial density, enzyme activity, phosphocreatine storage, and metabolic utilization of fuel sources.
By understanding the dominant energy system during specific exercise durations and intensities, individuals can create personalized training programs that target specific energy systems to optimize energy production and utilization. This knowledge allows for improved performance, enhanced endurance, and better overall fitness outcomes.
In conclusion, grasping the intricacies of energy system utilization during high-intensity exercise empowers individuals to fine-tune their training strategies, fueling techniques, and performance goals. Whether seeking to excel in explosive movements, endurance activities, or a combination of both, a comprehensive understanding of energy systems is crucial for unlocking peak athletic performance and achieving desired fitness results.