Home>Misc>Featured>What Type Of Fermentation Created In Humans From Long Distance Running Is
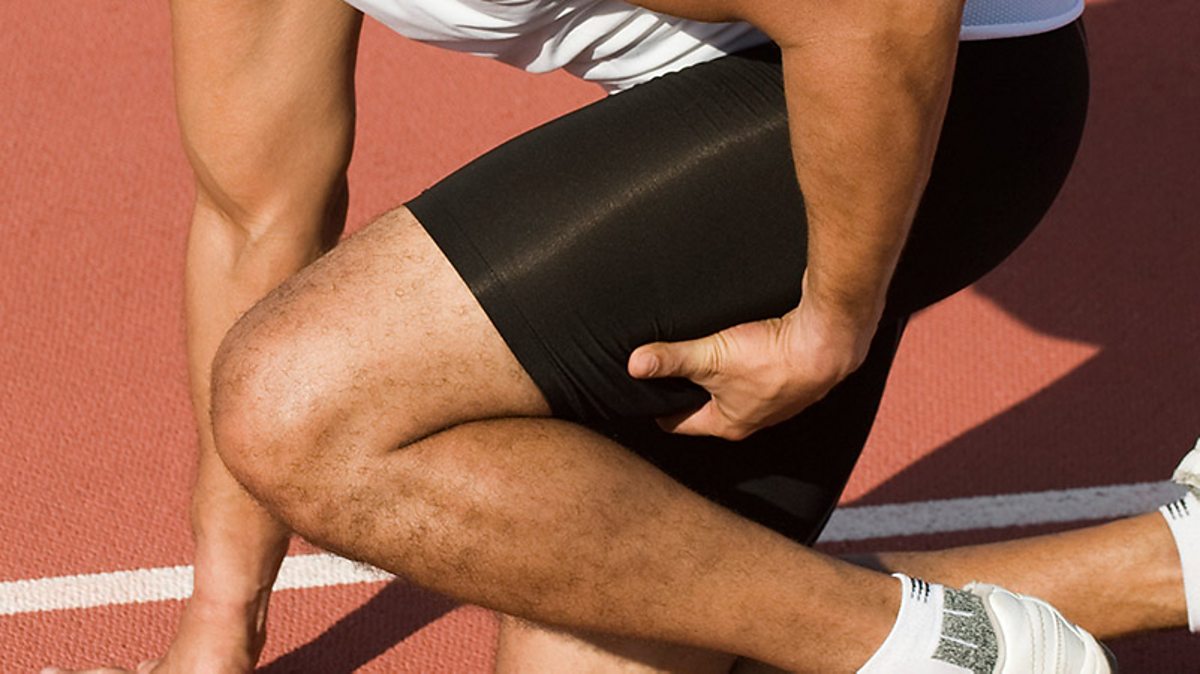
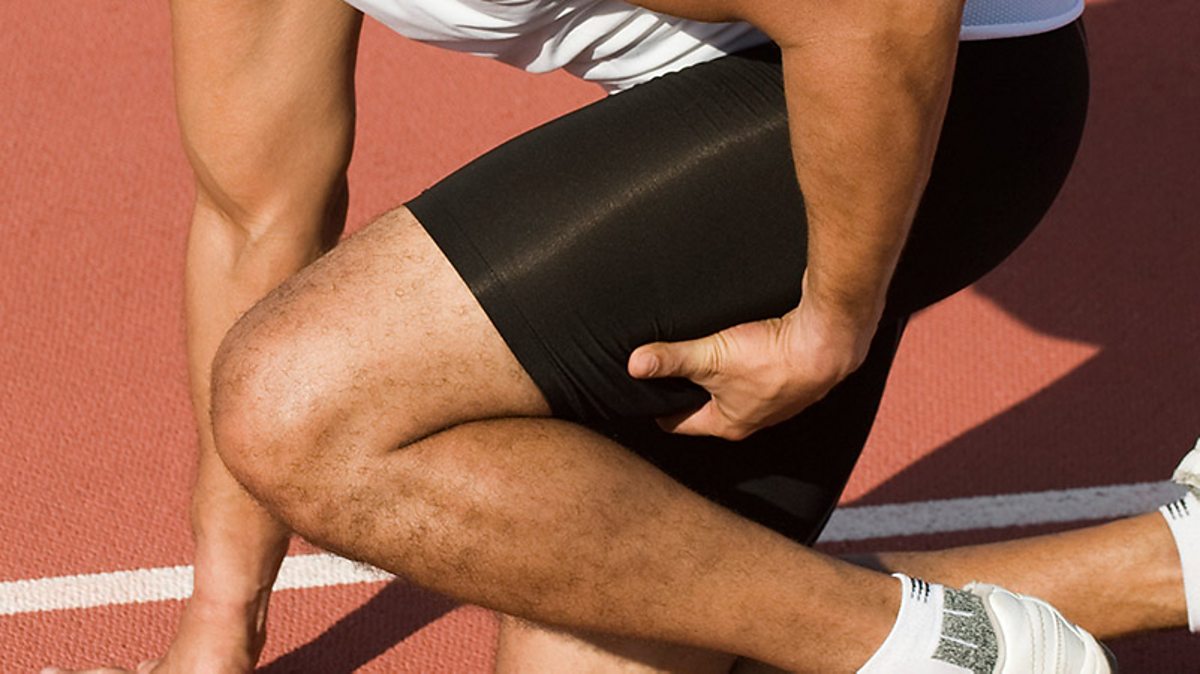
Featured
What Type Of Fermentation Created In Humans From Long Distance Running Is
Modified: January 2, 2024
Discover how long-distance running creates a unique type of fermentation in the human body. Gain insight into the featured process and its impact on athletic performance.
Introduction
The human body is a complex machine capable of performing remarkable feats of endurance and stamina. One such activity that pushes the limits of human performance is long-distance running. Whether it be marathons, ultramarathons, or other endurance races, these events test the physical and mental strength of athletes.
During intense exercise, the body undergoes various physiological changes to cope with the increased demands placed upon it. One notable process that occurs during long-distance running is fermentation. While fermentation is commonly associated with the production of alcoholic beverages and the making of bread, it also plays a significant role in the human body’s energy production during exercise.
In this article, we will explore the types of fermentation that occur in humans as a result of long-distance running. We will examine the connection between long-distance running and fermentation and discuss the benefits and consequences of these processes. By understanding the underlying mechanisms at play, athletes and fitness enthusiasts can optimize their training and improve performance.
It is important to note that while fermentation is typically associated with microorganisms, the focus of this article is on the metabolic processes that occur within the human body during exercise. Specifically, we will explore lactic acid fermentation and its effects on performance and fatigue, as well as briefly touch on other types of fermentation that may occur.
Now, let’s delve into the fascinating world of fermentation in humans and its impact on long-distance running.
Definition of fermentation in humans
Fermentation is a metabolic process that occurs in the absence of oxygen, allowing cells to generate energy in the form of adenosine triphosphate (ATP) without relying solely on aerobic respiration. In humans, fermentation occurs when the demand for energy exceeds the body’s capacity to supply it through oxygen-dependent processes, such as oxidative phosphorylation.
During intense exercise, the muscles require a constant supply of ATP to sustain contraction. However, the rate at which oxygen can be delivered to the muscles becomes limited, leading to an oxygen debt. In such situations, the body relies on fermentation as a means to continue producing ATP and meet the energy needs of the muscles.
Within the context of long-distance running, fermentation primarily refers to lactic acid fermentation, also known as anaerobic glycolysis. This process involves the breakdown of glucose or glycogen, stored forms of carbohydrates, to produce ATP and lactic acid. Unlike aerobic respiration, which occurs in the mitochondria and is highly efficient, lactic acid fermentation occurs in the cytoplasm of cells and is less efficient in terms of ATP production.
It is important to note that fermentation in humans is a temporary and adaptive response to meet the immediate energy demands of the muscles. Once oxygen becomes available in sufficient amounts, the body will switch back to aerobic metabolism to efficiently generate ATP. Therefore, fermentation in the context of exercise is a dynamic, transient process that reflects the body’s ability to adapt and meet energy requirements.
Now that we have established the definition of fermentation in humans, let’s explore the connection between long-distance running and these metabolic processes.
The connection between long-distance running and fermentation
Long-distance running is an endurance activity that places significant demands on the body’s energy systems. As the distance increases and the duration of the exercise extends, the reliance on aerobic metabolism becomes challenging due to limited oxygen availability. This creates a shift towards anaerobic metabolism, leading to an increase in fermentation processes within the body.
During long-distance running, the muscles contract repeatedly, requiring a continuous supply of ATP. As the intensity of the exercise increases, the body’s ability to deliver sufficient oxygen to the muscles becomes limited. As a result, the muscles turn to anaerobic metabolism, primarily relying on lactic acid fermentation to produce ATP.
This connection between long-distance running and fermentation can be understood through the principle of exercise intensity and the limitations of oxygen delivery. When running at a high intensity, the muscles require energy at a rapid rate, outpacing the oxygen supply. The body responds by utilizing fermentation processes to generate ATP more quickly, allowing the muscles to continue functioning.
Furthermore, the length of the running activity also plays a role in the connection to fermentation. In shorter-duration runs, the body relies primarily on aerobic metabolism to meet energy demands. However, as the duration of the run increases, the reliance on anaerobic metabolism and subsequent fermentation becomes more prominent.
It is important to note that the body’s reliance on fermentation during long-distance running is not exclusive to elite athletes or highly trained individuals. Even recreational runners experience some degree of fermentation when pushing themselves to the limits during endurance events. However, the extent of the reliance on fermentation may vary depending on factors such as training level, physical fitness, and individual physiology.
Understanding the connection between long-distance running and fermentation provides valuable insights into how the body adapts and sustains energy production during prolonged exercise. With this knowledge, athletes and trainers can develop strategies to optimize performance and mitigate negative impacts associated with fatigue and muscle acidosis.
Now that we have explored the connection between long-distance running and fermentation, let’s delve into the specific types of fermentation observed in humans during these activities.
Types of fermentation observed in humans during long-distance running
During long-distance running, the human body undergoes various types of fermentation to meet the energy demands of the muscles. The two most prominent forms of fermentation observed in humans during these activities are lactic acid fermentation and alcoholic fermentation.
1. Lactic Acid Fermentation:
Lactic acid fermentation, also known as anaerobic glycolysis, is the predominant form of fermentation observed during intense exercise. This process involves the conversion of glucose or glycogen into lactate, producing ATP in the absence of oxygen. Lactic acid fermentation occurs in the cytoplasm of cells and plays a crucial role in providing quick energy for muscle contraction during high-intensity activities like long-distance running.
2. Alcoholic Fermentation:
While lactic acid fermentation is the primary form of fermentation during exercise, there have been limited observations of alcoholic fermentation in humans during long-distance running. Alcoholic fermentation involves the conversion of glucose into alcohol and carbon dioxide and is commonly associated with the production of alcoholic beverages. However, compared to lactic acid fermentation, this type of fermentation is less significant in terms of energy production during exercise.
It is important to note that the specific types and extent of fermentation observed during long-distance running may vary among individuals. Factors such as training status, fitness level, and muscle fiber composition can influence the reliance on different fermentation pathways.
Understanding the types of fermentation that occur during long-distance running provides valuable insights into the metabolic adaptations that take place within the body. By optimizing training strategies and nutrition, athletes can enhance their ability to produce energy through fermentation, leading to improved performance and delayed onset of fatigue.
In the next section, we will delve deeper into the process of lactic acid fermentation, its role in energy production, and its effects on performance and fatigue during long-distance running.
Lactic Acid Fermentation
Lactic acid fermentation, also known as anaerobic glycolysis, is a metabolic process that occurs in the absence of oxygen during intense exercise. It plays a crucial role in meeting the energy demands of the muscles, particularly during long-distance running.
During lactic acid fermentation, glucose or glycogen is broken down into pyruvate through a series of biochemical reactions. Pyruvate is then converted to lactate by the enzyme lactate dehydrogenase. This process generates ATP, providing a rapid and efficient source of energy for muscle contraction.
Lactic acid fermentation is essential in situations where oxygen supply is limited, such as high-intensity exercise. As the demand for ATP outpaces the oxygen delivery to the muscles, the body shifts from aerobic metabolism to anaerobic metabolism. This shift allows for the rapid production of ATP despite the lack of oxygen.
One of the distinctive features of lactic acid fermentation is the accumulation of lactate in the muscles and bloodstream. The presence of lactate is often associated with muscle fatigue and the burning sensation experienced during intense exercise. However, it is important to note that lactate itself is not necessarily a waste product but rather serves as an important intermediate in the energy production process.
Contrary to popular belief, lactate does not directly cause fatigue. Instead, it acts as a fuel source for other tissues in the body, including the heart and liver. Lactate produced in the muscles during exercise can be transported to other tissues through the bloodstream and converted back into pyruvate, which can then be further metabolized for energy.
Furthermore, lactic acid fermentation plays a crucial role in replenishing energy stores during recovery periods. After intense exercise, the body continues to metabolize lactate, converting it back into glucose or glycogen through a process called the Cori cycle. This recycling of lactate helps replenish glycogen stores, ensuring a continuous supply of energy for subsequent bouts of exercise.
While lactic acid fermentation is an efficient way to produce ATP during intense exercise, it does have limitations. The process yields fewer ATP molecules compared to aerobic metabolism, leading to a buildup of metabolic byproducts such as hydrogen ions, which contribute to muscle soreness and fatigue.
Understanding the intricacies of lactic acid fermentation is crucial for optimizing performance during long-distance running. By implementing training strategies that improve the body’s ability to tolerate and efficiently utilize lactate, athletes can enhance their endurance and delay the onset of fatigue.
In the following sections, we will explore the production of ATP through lactic acid fermentation, as well as the effects of this process on performance and fatigue during long-distance running.
Production of ATP through lactic acid fermentation
ATP, or adenosine triphosphate, is the primary source of energy for cellular processes in the body, including muscle contraction during long-distance running. Lactic acid fermentation plays a key role in the production of ATP when oxygen availability is limited, as it provides a quick and efficient way to generate energy.
During lactic acid fermentation, glucose or glycogen is metabolized through a series of biochemical reactions, ultimately leading to the production of ATP. The initial step of glycolysis breaks down glucose into two molecules of pyruvate. Then, pyruvate is converted into lactate by the enzyme lactate dehydrogenase.
The conversion of pyruvate to lactate serves two primary purposes in ATP production. First, it helps regenerate NAD+, which is necessary for glycolysis to continue. By producing lactate, the lactate dehydrogenase enzyme allows glycolysis to proceed, ensuring the continued production of ATP in the absence of oxygen.
Second, the conversion of pyruvate to lactate generates two ATP molecules directly. This ATP production occurs through the transfer of high-energy phosphate groups from phosphoenolpyruvate to ADP, facilitated by the enzyme pyruvate kinase.
It is important to note that while lactic acid fermentation generates ATP, the total ATP yield is lower compared to aerobic metabolism. This is mainly due to the inefficient use of glucose or glycogen and the limitation of ATP production in the absence of oxygen.
However, the advantage of lactic acid fermentation lies in its ability to rapidly produce ATP. This is especially crucial during high-intensity exercise, such as long-distance running, where a rapid and immediate energy supply is required to sustain muscle contraction.
The production of ATP through lactic acid fermentation provides a valuable source of energy during intense exercise, allowing athletes to maintain performance despite limited oxygen availability. However, it is important to note that reliance on lactic acid fermentation for prolonged periods can lead to the accumulation of lactate and the onset of muscle fatigue.
The next section will explore the effects of lactic acid fermentation on performance and fatigue during long-distance running, shedding light on the importance of understanding and optimizing this metabolic process.
Effects of lactic acid fermentation on performance and fatigue
Lactic acid fermentation, while providing a quick source of ATP production, can have significant effects on performance and fatigue during long-distance running. Understanding these effects is crucial for athletes aiming to optimize their training and improve endurance.
One prominent effect of lactic acid fermentation is the onset of muscle fatigue. As the muscles rely more heavily on anaerobic metabolism and lactic acid production increases, there is a buildup of metabolic byproducts, including lactate and hydrogen ions. The accumulation of lactate can contribute to muscle acidosis, leading to a burning sensation and muscle discomfort during high-intensity exercise.
Moreover, the accumulation of lactate and hydrogen ions can impair muscle contractility and lead to a decrease in force production. This can manifest as a decrease in running speed, an inability to maintain pace, or a feeling of muscle weakness, ultimately impacting overall performance.
However, it is important to note that lactate is not solely responsible for muscle fatigue. Recent research suggests that lactate itself can serve as an additional fuel source for other muscles and organs in the body, including the heart and liver. In fact, lactate produced by the muscles during exercise can be transported through the bloodstream and used as an energy substrate by these tissues.
Additionally, lactate can act as a signaling molecule, triggering molecular and physiological adaptations that help improve performance. These adaptations include an increased recruitment of fast-twitch muscle fibers, improved buffering capacity, and enhanced lactate clearance mechanisms. Over time, these adaptations can lead to improved endurance and a decreased reliance on lactic acid fermentation.
Optimizing the effects of lactic acid fermentation on performance involves several strategies. Endurance training programs that emphasize both aerobic and anaerobic components can help improve lactate tolerance and clearance mechanisms. This type of training includes intervals, tempo runs, and long steady-state runs, allowing the body to become more efficient in utilizing lactate as a fuel source.
Furthermore, proper nutrition and hydration play a crucial role in supporting lactic acid fermentation and minimizing its negative effects. Consuming carbohydrates before, during, and after exercise can help maintain glucose levels and delay the onset of muscle fatigue. Hydration is also essential for optimal muscle function and lactate clearance.
By understanding and managing the effects of lactic acid fermentation, athletes can enhance their performance during long-distance running and delay the onset of fatigue. Through appropriate training, nutrition, and recovery strategies, individuals can optimize their energy production pathways and improve their overall endurance.
In the next section, we will briefly touch upon other types of fermentation that may occur in the human body during long-distance running, highlighting the importance of further research in this area.
Other types of fermentation observed in humans during long-distance running
In addition to lactic acid fermentation, there may be other types of fermentation observed in humans during long-distance running. While lactic acid fermentation is the primary form of fermentation during intense exercise, limited evidence suggests the presence of alternative pathways of fermentation within the body.
One potential form of fermentation that may occur during long-distance running is mixed acid fermentation. This type of fermentation involves the production of various organic acids, such as acetic acid, propionic acid, and butyric acid. These organic acids are byproducts of the breakdown of carbohydrates and can contribute to the overall metabolic response during exercise. However, further research is needed to fully understand the extent and significance of mixed acid fermentation in humans during long-distance running.
Another type of fermentation that has been observed in muscle cells during exercise is alanine fermentation. Alanine is an amino acid that can be produced through the transamination of pyruvate. During high-intensity exercise, muscles can release alanine into the bloodstream, where it can be converted back into glucose in the liver through a process called gluconeogenesis. This allows for the recycling of lactate and the replenishment of glucose stores, contributing to the overall energy balance during long-distance running.
It is important to note that the occurrence and impact of these alternative forms of fermentation during long-distance running may vary among individuals and depend on various factors such as training status, intensity of exercise, and individual physiology. Further research is needed to fully understand the significance and implications of these fermentations in the context of endurance exercise.
By gaining more insights into the various types of fermentation that may occur during long-distance running, researchers and athletes can further optimize training strategies, nutrition plans, and recovery techniques to enhance performance. Understanding the metabolic adaptations that take place within the body during exercise can lead to targeted interventions and improvements in endurance and overall athletic performance.
In the next section, we will discuss the importance of understanding fermentation in long-distance running and its implications for athletes and fitness enthusiasts.
Alcoholic Fermentation
Although lactic acid fermentation is the primary form of fermentation during exercise, there have been limited observations of alcoholic fermentation in humans during long-distance running. Alcoholic fermentation involves the conversion of glucose into alcohol and carbon dioxide and is commonly associated with the production of alcoholic beverages. However, compared to lactic acid fermentation, this type of fermentation is less significant in terms of energy production during exercise.
In alcoholic fermentation, glucose undergoes a series of reactions that convert it into ethanol and carbon dioxide. These reactions are facilitated by enzymes such as alcohol dehydrogenase. While alcoholic fermentation does occur to a small extent during exercise, its contribution to ATP production is minimal compared to lactic acid fermentation.
One reason for the limited occurrence of alcoholic fermentation in humans during long-distance running is the availability of oxygen. Even during intense exercise, there is usually a partial supply of oxygen, allowing for the continuation of aerobic metabolism. As a result, the body primarily relies on lactic acid fermentation to produce ATP, as it can provide energy more rapidly in the absence of sufficient oxygen.
It is important to mention that the presence of alcoholic fermentation in the human body during exercise is still a topic of ongoing research. Some studies have reported trace amounts of ethanol production in certain individuals, while others have not observed it at all. The variability in findings suggests that individual differences and other factors may influence the occurrence and extent of alcoholic fermentation.
While the significance of alcoholic fermentation in humans during long-distance running may be limited, understanding the metabolic processes involved is still valuable. Research in this area can shed light on the complexities of cellular metabolism and the potential interplay between different fermentation pathways within the human body.
However, it is essential to recognize that the primary focus in understanding and optimizing energy production during long-distance running lies in lactic acid fermentation. By improving lactate tolerance, clearance mechanisms, and overall metabolic efficiency, athletes can enhance their performance and endurance.
Now that we have explored the different types of fermentation observed in humans during long-distance running, let’s move on to discuss the importance of understanding fermentation in this context and its implications for athletes and fitness enthusiasts.
Importance of understanding fermentation in long-distance running
Understanding the various forms of fermentation that occur in the human body during long-distance running is essential for athletes and fitness enthusiasts. It provides valuable insights into the metabolic adaptations and energy production processes that take place during intense exercise, ultimately contributing to improved performance and endurance.
One of the key benefits of understanding fermentation in long-distance running is the ability to optimize training strategies. By knowing the predominant forms of fermentation, athletes can tailor their workouts to improve their tolerance to lactate and enhance their body’s ability to utilize it as a fuel source. Incorporating interval training, tempo runs, and specific lactate clearance workouts can help athletes improve their performance and delay the onset of fatigue during long-distance races.
Furthermore, understanding fermentation can also guide nutritional strategies to support energy production during long-distance running. Consuming carbohydrates before, during, and after exercise is crucial for maintaining glucose levels and providing the necessary fuel for fermentation processes. Proper hydration is equally important to support adequate blood flow and lactate clearance, ensuring optimal muscle function and performance.
Moreover, by understanding fermentation, athletes can refine their recovery techniques to promote efficient lactate clearance and replenishment of energy stores. Implementing strategies such as active recovery, foam rolling, and adequate rest can help facilitate lactate removal and minimize muscle soreness, ultimately supporting faster recovery and improved training adaptation.
Understanding fermentation in the context of long-distance running also highlights the need for individualized training programs. Every athlete is unique, with varied levels of lactate tolerance and capacity for utilizing different energy pathways. Therefore, tailoring training programs to each individual’s strengths and weaknesses can result in more effective and specific adaptations, leading to enhanced performance.
Additionally, advancements in research on fermentation and its implications for long-distance running can contribute to the development of innovative training methodologies and performance enhancement techniques. It can open doors to new training interventions, nutritional strategies, and recovery protocols that can push the boundaries of human endurance and improve overall athletic performance.
In summary, understanding fermentation in long-distance running is crucial for optimizing performance, improving energy production, and delaying the onset of fatigue. It enables athletes and fitness enthusiasts to tailor their training, nutrition, and recovery strategies specifically to the demands of their sport. By continuously expanding our knowledge of these metabolic processes, we can unlock new insights and approaches to maximize endurance and reach new milestones in long-distance running.
Conclusion
Long-distance running is a demanding endurance activity that relies on the body’s ability to produce energy efficiently. Fermentation, particularly lactic acid fermentation, plays a significant role in meeting the energy demands of the muscles during intense exercise. By understanding the types of fermentation that occur in the human body during long-distance running, athletes and fitness enthusiasts can optimize their training, nutrition, and recovery strategies to enhance performance and delay the onset of fatigue.
Lactic acid fermentation provides a rapid source of ATP production when oxygen availability is limited. It helps sustain muscle contraction and maintain energy supply during high-intensity exercise, such as long-distance running. While lactate accumulation has been associated with muscle fatigue, it is not solely responsible for fatigue and can serve as a fuel source for other tissues. The effects of lactic acid fermentation are further influenced by factors such as lactate tolerance, clearance mechanisms, and individual physiology.
Other forms of fermentation, such as alcoholic fermentation, may occur to a lesser extent during long-distance running. While their significance in energy production is minimal, continued research in this area helps unravel the complexities of cellular metabolism and the interplay between various fermentation pathways within the human body.
Understanding fermentation in the context of long-distance running allows for the development of targeted training programs, optimized nutrition plans, and effective recovery techniques. Individually tailored approaches can improve lactate tolerance, enhance energy production, and support faster recovery, leading to improved performance and endurance.
Furthermore, continued research in the field of fermentation and its implications for long-distance running can drive innovation and advancements in training methodologies, nutritional strategies, and performance enhancement techniques. It holds the potential to push the limits of human endurance and provide athletes with new opportunities for growth and achievement.
In conclusion, the deep dive into the world of fermentation in humans during long-distance running reveals the intricate mechanisms that enable the body to sustain energy production under challenging conditions. By harnessing our understanding of different fermentation processes and their effects, athletes can optimize their performance, enhance endurance, and reach new levels of success in long-distance running.